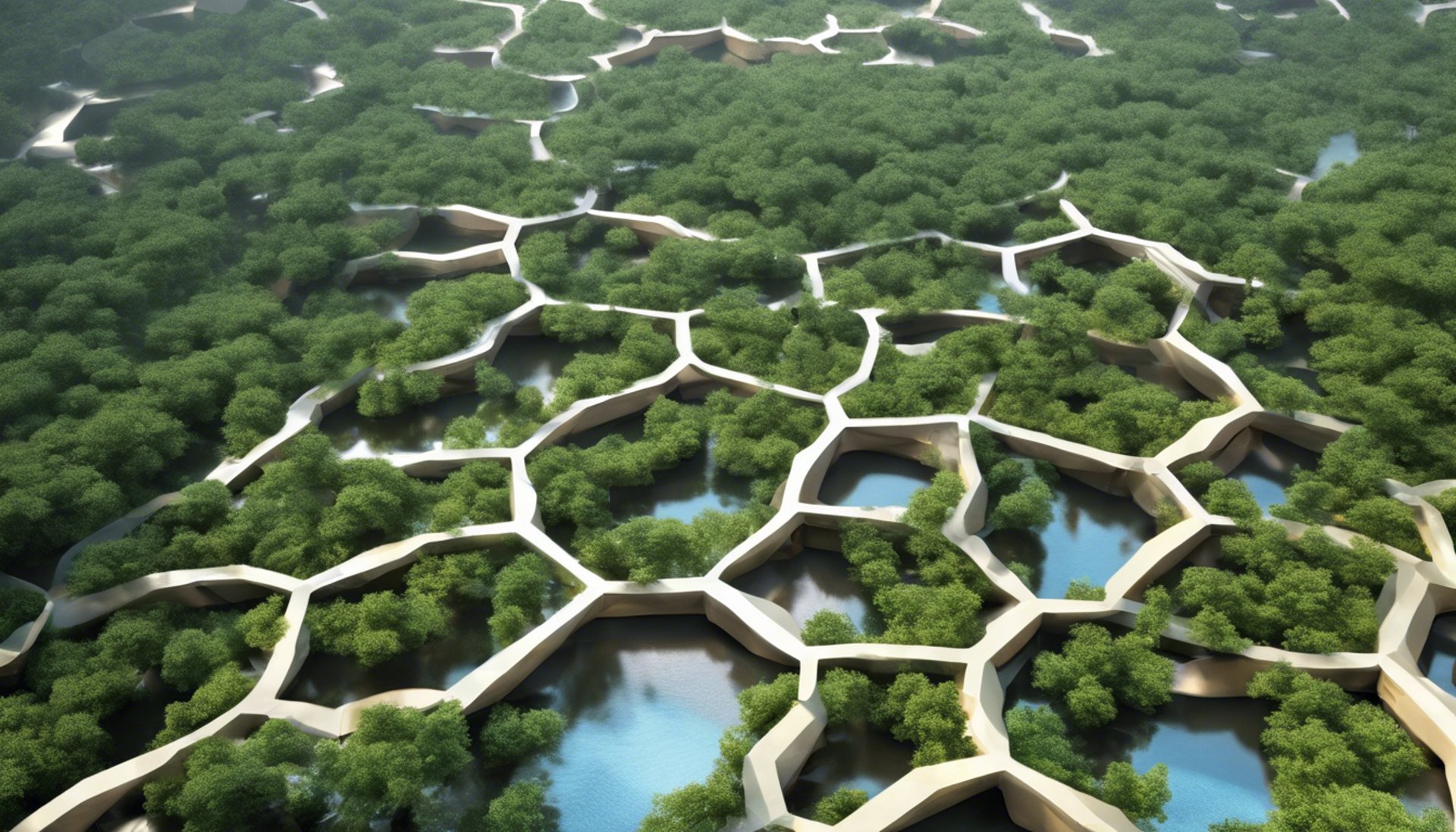
ACTION
IT’S TIME FOR
Sustainable Agriculture Land Management (SALM)
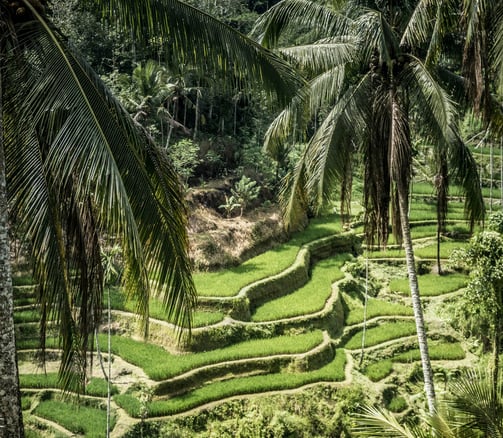
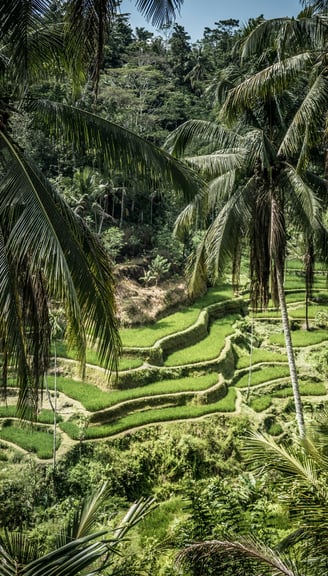
SALM refers to practices and techniques that prioritize the long-term health, productivity, and biodiversity of agricultural land while minimizing environmental impacts.
LAND MANAGEMENT FOR SUSTAINABLE AGRICULTURE (SALM): "SALM," describes methods and approaches that minimize environmental effects while placing an emphasis on the agricultural land's long-term health, productivity, and biodiversity. The following are examples of SALM practices:
Integrated Soil Fertility Management/Nutrient Management;
Conservation of Soil and Water;
Sustainable Agronomic Practices/Agroecological Practices;
Agroforestry;
Tillage and residual management;
Rehabilitation and restoration of degraded ecosystems, such as catchments;
Utilizing Sustainable Energy, such as biogas and solar;
Integrated Pest and Disease Management
SALM strives to preserve soil by minimizing erosion, enhancing soil fertility, and upholding soil structure by:
Water use optimization: Conserve water quality, minimize waste, and use resources wisely.
Promote biodiversity by preserving natural habitats, supporting beneficial creatures, and maintaining ecosystem services.
Improve ecosystem services by aiding with pest management, pollination, and climate regulation.
Boost crop yields: Boost output, cut losses, and improve food security.
Support rural livelihoods: Increase earnings, promote sustainable agriculture-based livelihoods, and boost rural well-being.
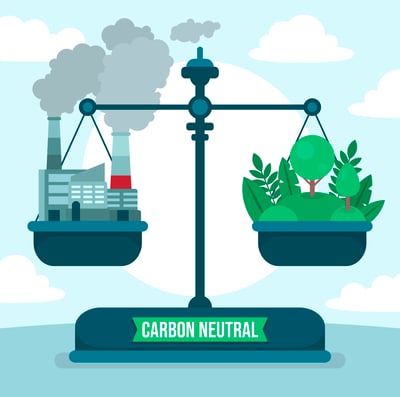
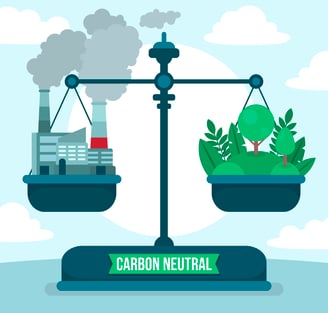
Scope 1, 2, and 3 GHG emissions
Scope 1
Direct Greenhouse Gas Emissions: Emissions originating directly from sources that are under the ownership or control of an entity.
Examples include: Emissions from chemical production processes; Fugitive emissions from on-site industrial activity (e.g., pipeline leaks); * Burning fossil fuels (natural gas, gasoline, or diesel) in on-site boilers, furnaces, or automobiles
Calculation:
Step 1: Establish the kind and amount of fuel (for example, cubic meters of natural gas or gallons of gasoline).
Step 2: Calculate emissions using emission factors, which are grams of greenhouse gas per unit of fuel. Sources such as the EPA and the Intergovernmental Panel on Climate Change (IPCC) provide emission factors.
Step 3: To determine the total emissions, multiply the fuel quantity by the emission factor.
Scope 2
Indirect Greenhouse Gas Emissions: The emissions resulting from the purchase of heat, steam, electricity, or air conditioning.
Examples include: Emissions from the power plants that supply the electricity a business buys; Emissions from the facilities that provide the heat or steam a business needs.
Calculation:
Step 1: Find out how much heat, steam, or electricity was bought.
Step 2: To estimate emissions, use emission factors (grams of GHG per unit of heat, steam, or electricity). These variables may change based on the area and energy source.
Step 3: To determine the total emissions, multiply the amount that was purchased by the emission factor.
Scope 3
Other Indirect Greenhouse Gas Emissions: These are emissions related to an organization's operations that take place outside of its direct control.
Examples include: Emissions from the manufacture of purchased materials; Emissions from the transportation of purchased materials or products; and Emissions from the usage of the organization's offered goods or services.
Calculation: Scope 3 emissions can be more difficult to quantify and frequently require gathering information from suppliers and other outside parties. Scope 3 estimates can be aided by a number of approaches and resources, including the GHG Protocol Corporate Accounting and Reporting Standard.
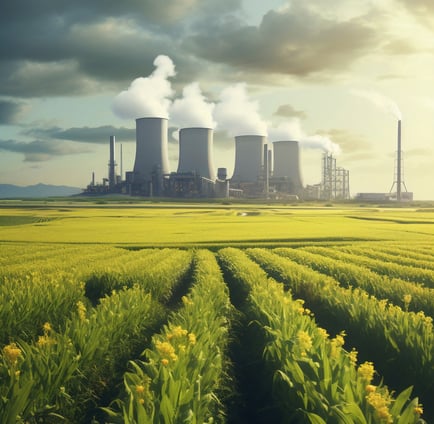
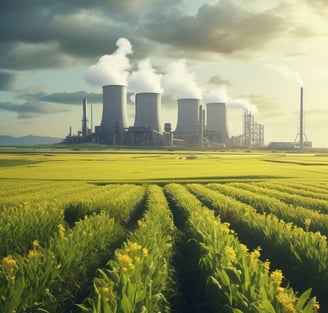
Green Ammonia- Combustion in Industrial Furnaces (use-case)
A clean, carbon-free fuel with a high hydrogen concentration that is perfect for industrial heating processes is emerging: green ammonia. Although issues like NOx emissions still exist, its potential is in its ability to be produced using renewable energy sources and in its adaptability for high-temperature applications.
Important developments in combustion technology include:
Novel injection methods that improve fuel-air mixing.
Enhanced functionality: Effectively regulate temperature.
Innovative furnace designs: Boost the effectiveness of combustion.
Strategies for reducing emissions: Try to reduce the production of NOx.
Difficulties with emissions:
NOx emissions: Demand cutting-edge methods for control.
Improved combustion techniques are required for unburned ammonia.
Mitigation strategies: Pay particular attention to safer burn technologies and following rules.
Industrial Impact:
While Europe, the US, and Australia are adopting and advancing sustainable solutions, Asia is leading research efforts in ammonia combustion.
Efficient & Emissions Balance:
bigger furnaces and swirl technologies
Efficient & Emissions Balance:
Swirl stabilization and gasification, two larger furnace technologies, improve combustion and lower emissions. Cutting NOx requires the use of SCR and SNCR.
Prospects for the Future:
Greener industrial heat requires widespread adoption of ammonia combustion, more efficiency, and tighter emission regulations. Sustainable development and innovation will be fueled by international cooperation.
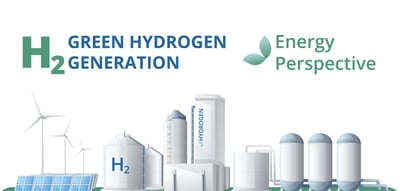
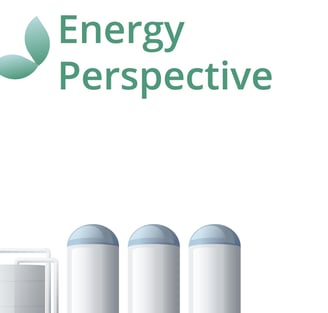
How the Green Hydrogen Ecosystem Works?
Production
Electrolysis:
Electrolysis is a method of producing green hydrogen by splitting water (H2O) into hydrogen (H₂) and oxygen (O₂) using electricity. To qualify the hydrogen as "green," the electricity utilized in this process needs to originate from renewable resources (such as hydro, solar, or wind power).
Renewable Energy: In order to guarantee environmentally friendly hydrogen generation, electrolysis electricity is produced solely from renewable sources, circumventing the need for fossil fuels. This indicates that every step of the process—from producing hydrogen to generating electricity—is low-carbon.
Transport and Storage
Storage: Generally, hydrogen is kept in chemical compounds such as metal hydrides, liquefied at extremely low temperatures, or high-pressure tanks. There are advantages and disadvantages to each approach in terms of cost and energy density.
Transport: Hydrogen can be moved as a liquid, in the form of chemical carriers, or via pipes. The infrastructural needs and efficiency of each technique vary.
Usage
Use of Fuel Cells: Hydrogen combines with atmospheric oxygen in a fuel cell to generate energy, with water being the sole byproduct. Electric cars, fixed power generators, and other devices can be powered by this.
Industrial Use: Hydrogen finds application in several industrial processes such as the manufacturing of ammonia for fertilizers, refining, and the possible substitution of coal in the steelmaking process.
Benefits for Sustainability
1. Reduction of Greenhouse Gas Emissions
Zero Emissions at Point of Use (e.g. as fuel cells)
Decarbonizing Hard-to-Abate Sectors (e.g. heavy industry (e.g., steelmaking) and long-haul transportation (e.g., trucks, ships))
2. Energy Storage and Flexibility
Seasonal Storage (can store excess renewable energy generated during periods of high production)
Grid Stabilization (helping to manage fluctuations in renewable energy generation)
3. Diversification of Energy Sources
Reducing Reliance on Fossil Fuels (supporting the transition to a low-carbon energy system)
Local Energy Production (can be decentralized, allowing regions to produce their own renewable energy)
4. Economic Opportunities
Job Creation (areas such as manufacturing, infrastructure development, and research)
Industrial Growth (can stimulate growth in new industries and technologies)
5. Environmental Benefits
Reduced Pollution (can help reduce air pollutants like NOx and particulate matter by reducing usage of fossil fuels)
Conservation of Resources (e.g. in steelmaking can reduce the need for coal and other carbon-intensive materials.
Obstacles
Cost: At the moment, producing green hydrogen is more expensive than producing gray hydrogen, which comes from fossil fuels. To make green hydrogen more competitive, the costs of electrolyzers and renewable energy sources must come down.
Infrastructure: Creating the necessary planning and financial resources is necessary for the storage, distribution, and transportation of hydrogen.
Energy Efficiency: To optimize the overall efficacy of green hydrogen, the energy conversion efficiency of electrolysis, storage, and fuel cells must be increased.